Everything You Need to Know About Energy Systems
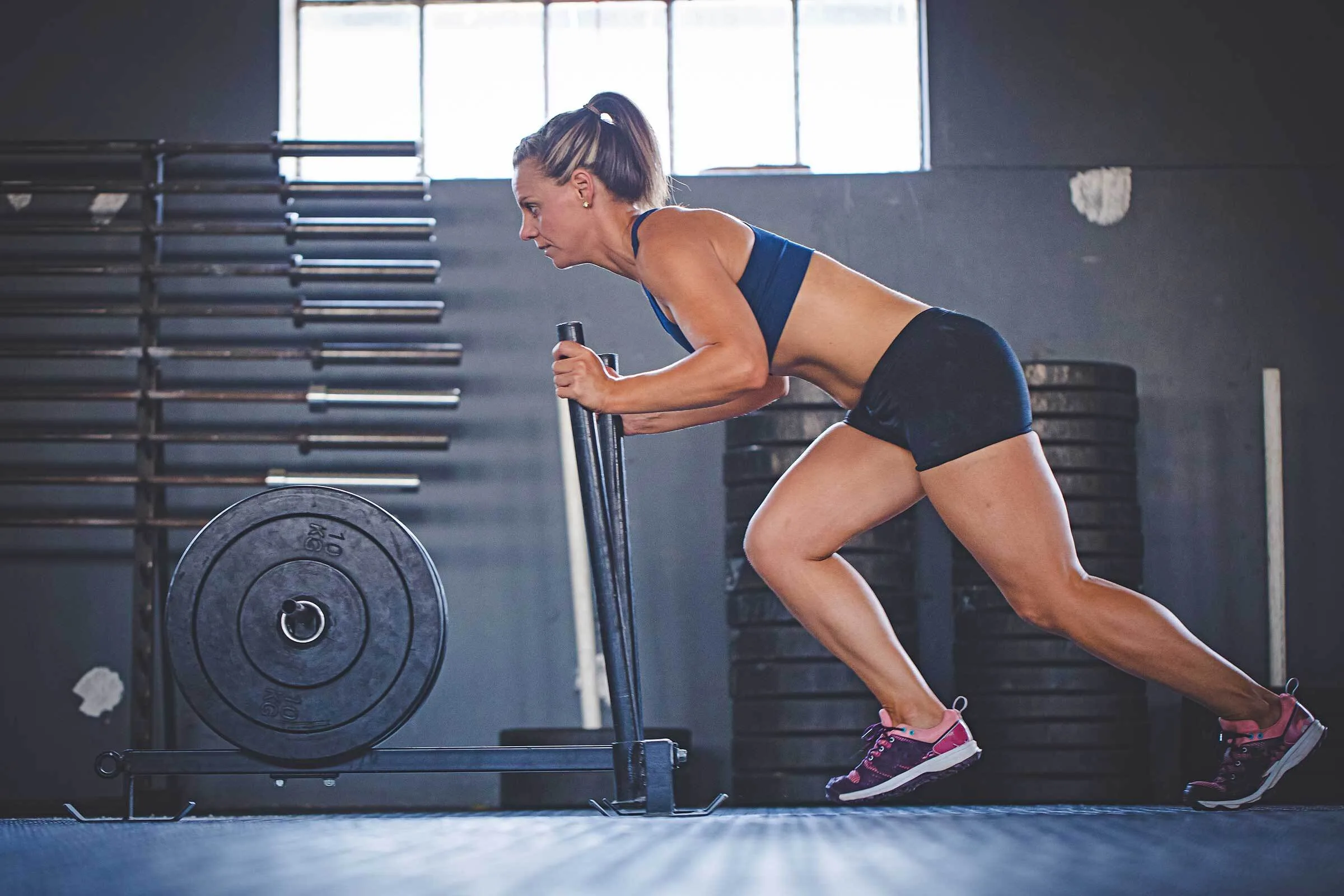
It pays to know about your body’s energy systems, whether you’re a coach or an athlete.
Jonathan Mike, PhD, CSCS*D, NSCA-CPT*D, USAW, NKT-1 is a currently a faculty member the Exercise Science department at Grand Canyon University in Phoenix, AZ. He is also a strength coach, author and speaker, and has competed in the sports of strongman and jujitsu. In this blog, he outlines the energy systems of the body and their relevance for coaches and athletes.
jonathan mike
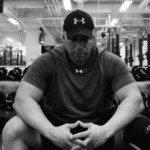

Change the Way You Train
The Truth About Energy Systems
Energy systems within the framework of coaching and athletic development is always a heated topic, and there has been this very unsettling trend within social media and online articles discussing the best methods to incorporate these systems into programs. While this topic is critical to the improvement of athletic performance, implementation, and program design, the use of specific terms and application have been misinterpreted.
As times goes on, the staggering amount of self-proclaimed training and nutrition “experts” within the industry become more obvious. Many are discussing and even advocating basic programming ideas based on these systems without ever really thinking about how they actually work. There are way too many people who speak and write as though these energy systems are completely independent of each other.
Here’s the truth – and I know it may be shocking – but all these energy systems are not independent of each other. They never have been and never will be.
This article will help set the record straight and examine the truth about energy systems, what they are, how they work, and what you really need to know. Let’s get started!
Energy Systems 101
The essential terminology governing energy systems is called bioenergetics. It’s the conversion of chemical energy to mechanical energy. In other words, the conversion of carbs, proteins, and fats (chemical energy) into biological energy (mechanical), or what you use during training sessions.
Our bodies are in a constant state of anabolism and catabolism (called metabolism), which is the sum of all the anabolic and catabolic reactions in the body.
Energy from catabolic reactions is used to drive anabolic reactions through, you guessed it, adenosine triphosphate (ATP). Without ATP, muscle activity and growth wouldn’t occur, plain and simple.
What is ATP?
ATP is made up adenosine (nitrogen base), ribose (five-carbon sugar), and three phosphate groups. Essentially, if you remove a phosphate group, ATP becomes diphosphate (ADP). Remove another and ADP becomes monophosphate (AMP).
Here’s the scoop—your cells can’t generate ATP from scratch.
If we think back to the first law of thermodynamics, the total amount of energy in the universe remains constant. Therefore, from the foods we eat, potential energy exists within cells in the chemical bonds such as glucose, glycogen (composed of hundreds or thousands of glucose molecules stored in the muscles, liver, and brain), and fatty acids. When these compounds enter energy pathways, some become rearranged with energy released and captured in the formation of ATP. This “new energy” is then used for cell functions such as supplying the energy for muscle contraction (like heavy ass lifting).
The energy for all these processes is liberated from ATP by removing the terminal inorganic phosphate (Pi) group from the molecule, leaving adenosine diphosphate (ADP) plus one proton (H+). This ADP is readily recycled in the mitochondria (power source organelle in cells) where it is renewed again to ATP.
ATP is broken down (hydrolysis) because water is the splitting molecule. ATP is not much of a storage fuel. Muscle cells store ATP only in limited amounts, and activity requires a regular supply, which provides energy needed for muscular work.
Anaerobic vs. Aerobic
Now, this is where things start to get misinterpreted and perhaps a little confusing. We have to briefly touch on this because this sets the stage for the actual terms for true energy systems discussed later.
Most people would say, “Aerobic metabolism is an energy generating reaction that ‘uses’ oxygen.” While this statement isn’t totally wrong, it does leave out some keys aspects. Oxygen actually serves as the final electron acceptor in the chain (electron transport chain) and combines with hydrogen to form water (13).
Now, the term “aerobic” may seem misleading because oxygen does not actually participate directly in ATP synthesis. Instead, it is present at the “end stage” and significantly determines the ability for aerobic ATP production and sustained exercise, particularly during endurance exercise (13) and throughout heavy strength training as well.
As we’ll see more in the next section for energy systems, research suggests that the oxidative system also works hard to help you recover after a high intensity anaerobic effort like a set of squats or hill sprints (4).
With anaerobic, the generation of high energy via quick, intense exercise bouts is facilitated through the immediate anaerobic system, which has no reliance placed on oxygen due to the nature of the stimulus and combined efforts of the other two high energy systems.
Some metabolic pathways require oxygen. They are said to be aerobic and will not proceed unless oxygen is present in sufficient concentrations.
Other processes don’t require oxygen to proceed to completion and are said to be anaerobic.
The important underlying message is that oxygen can play a pivotal role in some pathways and have little effect on others. However, this variation in cells is actually ideal so they can adapt to cellular energy needs (at least temporarily) independent of oxygen.
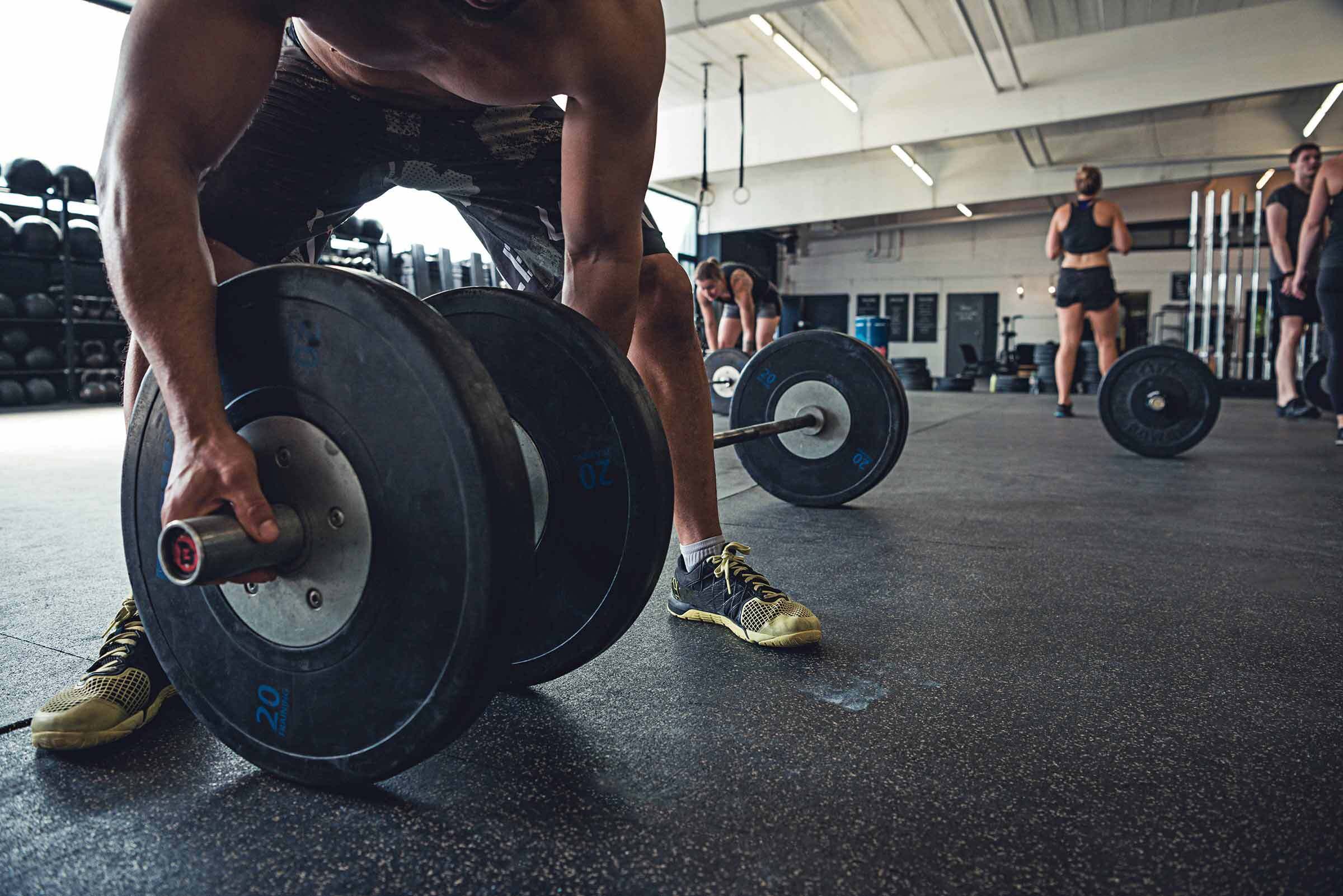
Energy Systems: The Correct Terms
The Phosphagen System
During short-term, intense activities, a large amount of power needs to be produced by the muscles, creating a high demand for ATP. The phosphagen system is the quickest way to resynthesize ATP and is active at the start of all types of training regardless of intensity (6). The creatine kinase (enzyme) reaction regulates the breakdown in creatine phosphate.
Here’s the scoop—at the start of training, ATP is broken down into ADP, releasing energy for muscle contractions. The increase in ADP activates the creatine kinease (enzyme) reaction to promote the formation of ATP from the breakdown of creatine phosphate.
If training continues at high intensities, this enzyme reaction (CK) remains elevated. Once training is over or continues at a low enough intensity, it will allow glycolysis or the oxidative (mitochondrial respiration) system to supply adequate ATP for muscle energy demand.
Because ATP and creatine phosphate are stored in the muscle in small quantities, the phosphagen system can’t supply enough energy for continuous, long duration training. Overall, type II (fast twitch) motor units contain greater concentration of phosphagens compared to type 1 (slow twitch) motor units (10). Creatine phosphate (CP) is stored in skeletal muscles and donates a phosphate to ADP to produce ATP. No carbohydrate or fat is used in this process. However, the regeneration of ATP exclusively comes from stored CP. This process does not require oxygen to resynthesize ATP because it is anaerobic or oxygen independent.
The phosphagen system is the predominant energy system used for all-out exercise lasting up to about ten seconds and sometimes fifteen seconds, although there is currently not an exact time frame. However, because there is a limited amount of stored CP and ATP in skeletal muscles, the onset of fatigue occurs quickly.
This is the primary energy pathway that creatine monohydrate supplementation is built upon. It’s highly effective in the rapid regeneration of ADP to ATP from the creatine kinase reaction during andfollowing intense exercise, primarily from resistance training. It is undoubtedly the most studied performance supplement in the last twenty years, as the body of evidence clearly supports that creatine enhances exercise capacity and performance (1, 2, 7, 9, 11).
Glycolysis
Glycolysis helps to supplement the phosphagen system initially and then becomes the primary ATP source during high intensity muscular work that lasts from thirty seconds to about 2–3 minutes. It is the second fastest way to resynthesize ATP. Glycolysis, which is the breakdown of glucose, is one of the most studied metabolic pathways in the exercise sciences.
During glycolysis, carbohydrates in the form of either blood glucose or muscle glycogen (the stored form of glucose) are broken down through a series of chemical reactions to form pyruvate (glycogen is first broken down into glucose through a process called glycogenolysis).
Now, at the end stage of glycolysis via the breakdown of glucose, you get two pyruvate and two ATP. Once pyruvate is formed, it can go two directions: conversion to lactate or conversion to acetyl coenzyme A (acetyl-CoA), which enters the mitochondria for oxidation and the production of more ATP (10).
Simply put, the conversion to lactate occurs when the demand for oxygen is greater than the supply (e.g., during anaerobic exercise via sprinting or high intensity resistance training). In contrast, when there is enough oxygen available to meet the muscular and metabolic demands (e.g., during aerobic exercise), pyruvate (via acetyl-CoA) enters the mitochondria and goes through aerobic metabolism.
When oxygen is not supplied fast enough to meet the demands of muscular effort, an increase in hydrogen ions occurs, causing muscle pH to decrease (called acidosis) and other metabolites. Acidosis and the accumulation of these other metabolites cause a number of issues that can significantly lower your performance and interfere with everything inside and outside of the muscle. Due to the loss of performance, muscles lose their ability to contract effectively, and muscle force production and exercise intensity are ultimately ceased.
Mitochondrial RespirationI (Aerobic System)
From these three energy systems, the aerobic system, which is dependent on oxygen, is the most complex (3, 16). The metabolic reactions that take place in the presence of oxygen are responsible for most of the cellular energy produced by the body. As most people know, aerobic metabolism is the slowest way to resynthesize ATP. It provides a large magnitude of ATP, but the tradeoff is that you have to wait for it.
This system includes the citric acid/Krebs cycle, or the better term TCA cycle. I like TCA because it sounds better and should be used more often.
This system also involves the electron transport chain, which simply uses blood glucose, glycogen, and fat as fuels to resynthesize ATP in the mitochondria of muscle cells. When using carbohydrates, glucose and glycogen are first metabolized through glycolysis, with the resulting pyruvate used to form acetyl-CoA, which enters the TCA. The electrons produced in the TCA are then transported through the electron transport chain where ATP and water are produced. This is technically termed oxidative phosphorylation (6, 10).
Thus, the aerobic system produces eighteen times more ATP than does anaerobic glycolysis from each glucose molecule.
What About Fat?
This is nearly another topic and probably a future article in itself, but essentially intramuscular triglyceride is the other major fuel for the aerobic system.
Fat oxidation also requires oxygen. Because fats are long carbon chains, they are transported to the muscle mitochondria where the carbon atoms are used to produce acetyl-CoA (a process called beta-oxidation). When using fat, triglycerides are first broken down into free fatty acids and glycerol (a process called lipolysis). The breakdown of fatty acid compounds goes directly into the mitochondrion to produce ATP, as the oxidation of free fatty acids generate considerable more ATP molecules than the oxidation of glucose or glycogen.
A Quick Recap
There are way too many people who speak and write as though these energy systems are completely independent of each other. All these energy systems are not independent of each other.
- Phosphagen system: Forming ATP by using creatine phosphate or two ADP molecules; this pathway yields the highest ATP turnover rate
- Glycolysis: From blood glucose or muscle glycogen; can activate very early on in muscle contraction
- Mitochondrial respiration: The use of oxygen in the mitochondria
Intense, sustained movements, such as a 400-meter race, are dependent on not only the phosphagen system, but also largely on a slower pathway of ATP production, glycolysis, and to a much smaller extent, the slowest pathway of producing ATP, mitochondrial respiration.
As the duration of maximal effort work is sustained, the absolute power output will decline, as there is a shift in the energy system that is most dominant in resynthesizing ATP. In this sense, energy systems represent a continuum that ranges from rapid energy production to slow energy production.
It is important to note that at any given time, all energy systems are in operation. While we often like to classify activities as anaerobic or aerobic, each energy system is making a contribution to the total energy production.
For example, if a person is performing a max effort squat, the majority of energy used to supply the contracting muscles is being derived from the phosphagen system and anaerobic glycolysis. However, what energy system would the arms be using? How about the heart? Perhaps the only time in which an animal would be in a true anaerobic state is during the final moment of life.
Intense, sustained movements, such as a 400-meter race, are dependent on not only the phosphagen system, but also largely on a slower pathway of ATP production, glycolysis, and to a much smaller extent, the slowest pathway of producing ATP, mitochondrial respiration.
As the duration of maximal effort work is sustained, the absolute power output will decline, as there is a shift in the energy system that is most dominant in resynthesizing ATP. In this sense, energy systems represent a continuum that ranges from rapid energy production to slow energy production.
It is important to note that at any given time, all energy systems are in operation. While we often like to classify activities as anaerobic or aerobic, each energy system is making a contribution to the total energy production.
For example, if a person is performing a max effort squat, the majority of energy used to supply the contracting muscles is being derived from the phosphagen system and anaerobic glycolysis. However, what energy system would the arms be using? How about the heart? Perhaps the only time in which an animal would be in a true anaerobic state is during the final moment of life.
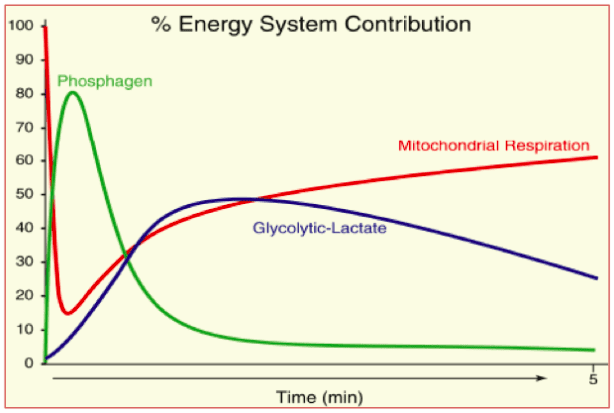
Practical Applications for the Energy Systems
Since all three of your energy systems ultimately run on ATP, let’s break it down by each energy system.
Phosphagen
Let’s say you’re doing a max effort squat, as you move the weight via the concentric phase, posterior chain musculature immediately burn through their ATP stores. Once the ATP has been used, it’s either further broken down or recycled via creatine phosphate (see above sections), so it can provide additional energy for the working muscles.
It’s the fuel source for all your physical functions, from eating to breathing to pushing the Prowler®.
Most people don’t realize how quickly the phosphagen system is activated; it simply takes thousandths of a second. Like many things, there is a benefit to risk ratio, or in the case of energy systems, a speed and efficiency cost.
The phosphagen system can only supply enough ATP and CP lasting for a minimum of 6 seconds, and up to 15 seconds of serious effort.
Despite the fact that the phosphagen system will enhance and provide explosive speed and power (e.g., shot put throw, Olympic weightlifting, max deadlift, vertical jump, 100m sprint, yoke walk, etc.), it does not afford the ability to operate at full throttle beyond the time frame (6-15 seconds). Further, the majority of trained athletes require three to five minutes of rest before their ATP is resynthesized and they can continue to perform near the level of their previous effort. (15)
Glycolysis
As the phosphagen system dwindles down (although it becomes re-synthesized), the glycolytic system becomes more activated for another several minutes before it begins to diminish too. As discussed above in more detail, glycolysis converts carbohydrates (glucose) into ATP. Although less sensitive compared to the phosphagen system, your glycolytic system continues to provide as much as half the energy in the first several seconds of intense training.
The best example of all-out glycolysis are 200-meter and 400-meter sprints. Usain Bolt ran a 400 in 46.74 seconds and recently won the world championships in the 200 in 19.66 seconds. That wasn’t even a true max run for him.
Despite what ignorant gurus and online coaches and trainers might tell you, the “burning sensation,” for lack of a better term, that you get when the exercise intensity is very high is not caused by lactic acid. In fact, there isn’t any such thing as lactic acid.
There is only lactate.
This is due to the buildup of hydrogen ions, a byproduct of glycolysis. In fact, accumulation of muscle and blood lactate after exercise can be oxidized back to pyruvate for gluconeogenic conversion of glucose in the liver or be converted back to pyruvate in the muscle for mitochondrial ATP production. It’s a fuel source. If the muscle did not produce or could not produce lactate, there would be no means to rely on additional ATP from glycolysis and no reaction would take place to regenerate energy.
Lactate is a good thing.
Further, the more you train your glycolytic system, the better you’re able to buffer these ions and the faster you can recover between sets of moderate to high intensity training. Of course, this is really the foundation for at least one factor of high intensity interval training (HIIT) that fitness professionals continue to recommend and incorporate into their programs for people who want to gain muscle, lose fat, and maximize results in their training, depending on their goals.
A study published this year showed that high intensity interval training (HIIT) performed in an “all-out” manner (e.g. repeated Wingate tests) is a time-efficient strategy to induce skeletal muscle remodeling toward more oxidative aspects of muscle. The training, which lasted less than ten minutes per session including the warm up and was performed three times a week for six weeks, improved chronic muscle adaptations (V02) in young healthy subjects (8).
In addition, glycolysis does very well in fat loss due to its metabolic stress. Recovering from it actually requires work from all three energy systems. Specifically, glycolytic training develops not only the operation of each individual system, but also the capability to transition efficiently between them. The primary methods to train your glycolytic system are through repeated high effort activities with less than full recovery between efforts via 20- to 30-second sprints with a minute of rest between them or strength training sets lasting thirty seconds to one minute (6, 10, 13).
Mitochondrial Respiration
Mitochondrial respiration, or your aerobic system, operates continuously and is probably the most important both at rest and during training. It is fueled primarily on fat and glucose. As previously mentioned in the first sections of this article, it’s the only system that directly requires oxygen in order to function.
Now, despite the fact that this energy system is ongoing, generally speaking, it’s the last system to kick in. However, highly trained athletes, particularly endurance athletes, have such a dominate oxidative system that it actually activates much sooner compared to normal individuals. These athletes are able to reach a steady state much quicker compared to less trained individuals. The majority of long distance events and sports (marathon, cycling) require exceptional aerobic capacity, as do athletes in all continuous action field and team sports (soccer, basketball).
Traditionally, the belief was that the best way to develop the oxidative system was through long, slow cardio exercise. Times have changed. Although many still do believe this and even though the aerobic system certainly responds well to that type of training, recent research suggests the oxidative system also works extremely hard to help you recover after high intensity anaerobic efforts (14).
Due to the intermittent nature of heavy strength training, the energy expenditure contributions of weight training are almost always in a non-steady state compared to aerobic type exercise, which is usually defined as being steady state.
What does this mean? Well, a resistance training session corresponds to a series of intermittent/non-steady state waves of intensity and effort for the oxidative system (mitochondrial respiration). Many times, individuals feel “gassed” after high intensity bouts of strength training or conditioning even though these specific bouts themselves are technically anaerobic.
What is happening is that mitochondrial respiration (oxidative system) moves into hyper-drive in order to replenish the depleted ATP stores and remove the buildup of metabolic byproducts that were produced from the other energy systems (phosphagen and glycolysis).
Upon the cessation of intense lifting or interval work, mitochondrial respiration (oxidative) continues activity for a few days. This is where the concept and application of excess post-exercise oxygen consumption (EPOC) comes in. This occurrence can burn additional fat and calories for up to 72 hours post-training when intensity and duration (especially intensity) are the presiding factors (5, 12).
Here’s the deal—unless you compete in endurance events, performing lots of long, slow cardio isn’t the best way to train or enhance your aerobic system. You are better off engaging in higher intensity training as a more effective and efficient means to develop your oxidative system and drive fat burning.
Lastly, because low intensity aerobic activity enhances recovery from other forms of training, perhaps the best use of oxidative training is a recovery tool used on non-training days.
References
Bemben MG, Lamont HS (2005) Creatine supplementation and exercise performance: recent findings. Sports Med 35:107–25.
Bemben MG, Witten MS, Carter JM, Eliot KA, Knehans AW, Bemben DA (2010) The effects of supplementation with creatine and protein on muscle strength following a traditional resistance training program in middle-aged and older men. The Journal of Nutrition, Health & Aging 14:155–59.
Bishop DJ, Granata C, Eynon N (2013) Can we optimize the exercise training prescription to maximize improvements in mitochondria function and content? Biochim Biophys Acta.
Bishop PA, Jones E, Woods AK (2008) Recovery from training: a brief review: brief review. J Strength Cond Res 22:1015–1024.
Borsheim E, Bahr R (2003) Effect of exercise intensity, duration and mode on post-exercise oxygen consumption. Sports Med 33:1037–1060.
Brooks G, Fahey Baldwin (2005) Exercise Physiology: Human Bioenergetics and Its Application.
Buford TW, Kreider RB, Stout JR, Greenwood M, Campbell B, Spano M, Ziegenfuss T, Lopez H, Landis J, Antonio J (2007) International Society of Sports Nutrition position stand: creatine supplementation and exercise. J Int Soc Sports Nutr 4:6.
Cochran AJ, Percival ME, Tricarico S, Little JP, Cermak N, Gillen JB, Tarnopolsky MA, Gibala MJ (2014) Intermittent and continuous high-intensity exercise induce similar acute but different chronic muscle training adaptations. Exp Physiol.
Jones AM, Atter T, Georg KP (1999) Oral creatine supplementation improves multiple sprint performance in elite ice-hockey players. J Sports Med Phys Fitness39:189–96.
Kraemer WJ, Fleck, SJ, Deschenes MR (2012) Exercise Physiology: Integrating Theory and Application. Philadelphia: Wolters Kluwer/Lippincott Williams & Wilkins.
Kreider RB (2003) Effects of creatine supplementation on performance and training adaptations. Mol Cell Biochem 244:89–94.
LaForgia J, Withers RT, Gore CJ (2006) Effects of exercise intensity and duration on the excess post-exercise oxygen consumption. J Sports Sci 24:1247–64.
McArdle K, Katch (2009) Exercise Physiology: Energy, Nutrition, and Human Performance.
Scott CB (2011) Quantifying the immediate recovery energy expenditure of resistance training. J Strength Cond Res 25:1159–63.
Weiss L (1991) The obtuse nature of muscular strength: The contribution of rest to its development and expression. Journal of Applied Sports Science Research5:219–27.
Yan Z, Lira VA, Greene NP (2012) Exercise training-induced regulation of mitochondrial quality. Exerc Sport Sci Rev 40:159–64.
Find Your Perfect Training Plan
Sometimes all you need to reach your destination on your fitness journey is an expert guide. We've got you covered. Browse from thousands of programs for any goal and every type of athlete.
Try any programming subscription free for 7 days!
Related articles
3 Ways to Improve Mobility Without Stretching
Are you still trying the endless foam rolling and stretching exercises to get that deep squat position? We know how important mobility is for great, or even GOOD performance. All professional athletes have some comfortability in end ranges of motion. So, what else do...
The Ultimate Guide to Lunges: Queen of all Glute Exercises
Your glutes are the largest muscle group in your body. They’re responsible for almost everything your legs do—walking, running, jumping, squatting, lunging, and just standing upright. As far as moving through space goes, strong glutes are the bedrock of overall...
A Beginner’s Guide to Steel Mace Training
Author: Jesse Grund
Mace training will make you a better mover without it’s not confining you to a fixed space or predetermined range of motion. Second, it’s an offset load with 80 to 90 percent of the weight in the head. You’re also constantly having to resist rotation, which creates greater core engagement.
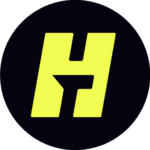
Join the community
Sign up for the latest training news and updates from TrainHeroic
Made with love, sweat, protein isolate and hard work in Denver, CO
© 2023 TrainHeroic, Inc. All rights reserved.